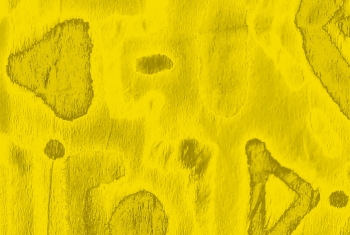
Crown nanoplatelets
In recent years, scientists have been experimenting with combining semiconductors at the nanoscale, creating new nanostructures with interesting optoelectronic properties. Among the different colloidal nanostructures that can be made are 2D nanoplatelets, named for their thin tray-like shapes when seen through an electron microscope. They combine strong and weak quantum confinement regimes--which, on the one hand, yield a band-edge emission peak at discrete wavelengths; and on the other hand, yield a large band-edge oscillator strength and fast emission lifetime that scales with the area of the nanoplatelet.
The thickness can be controlled with monolayer precision. They also exhibit a high, two-photon absorption coefficient, and the 2D shape leads to opportunities for self-assembly and ultrafast Förster energy transfer between the nanoplatelets. These properties allow them to be tuned for a variety of optoelectronic tasks.